Quantum Phenomena at Room Temperature
In the realm of quantum mechanics, the ability to observe and control quantum phenomena at room temperature has long been elusive, especially on a large or “macroscopic” scale. Traditionally, such observations have been confined to environments near absolute zero, where quantum effects are easier to detect. But the requirement for extreme cold has been a major hurdle, limiting practical applications of quantum technologies.
Now, a study led by Tobias J. Kippenberg and Nils Johan Engelsen at EPFL, redefines the boundaries of what’s possible. The pioneering work blends quantum physics and mechanical engineering to achieve control of quantum phenomena at room temperature.
“Reaching the regime of room temperature quantum optomechanics has been an open challenge since decades,” says Kippenberg. “Our work realizes effectively the Heisenberg microscope – long thought to be only a theoretical toy model.”
In their experimental setup, published in Nature, the researchers created an ultra-low noise optomechanical system – a setup where light and mechanical motion interconnect, allowing them to study and manipulate how light influences moving objects with high precision.
"These systems are useful for quantum information, and help us understand how to create large, complex quantum states." - Alberto Beccari
The main problem with room temperature is thermal noise, which perturbs delicate quantum dynamics. To minimize that, the scientists used cavity mirrors, which are specialized mirrors that bounce light back and forth inside a confined space (the cavity), effectively “trapping” it and enhancing its interaction with the mechanical elements in the system. To reduce the thermal noise, the mirrors are patterned with crystal-like periodic (“phononic crystal”) structures.
Another crucial component was a 4mm drum-like device called a mechanical oscillator, which interacts with light inside the cavity. Its relatively large size and design are key to isolating it from environmental noise, making it possible to detect subtle quantum phenomena at room temperature. “The drum we use in this experiment is the culmination of many years of effort to create mechanical oscillators that are well-isolated from the environment,” says Engelsen.
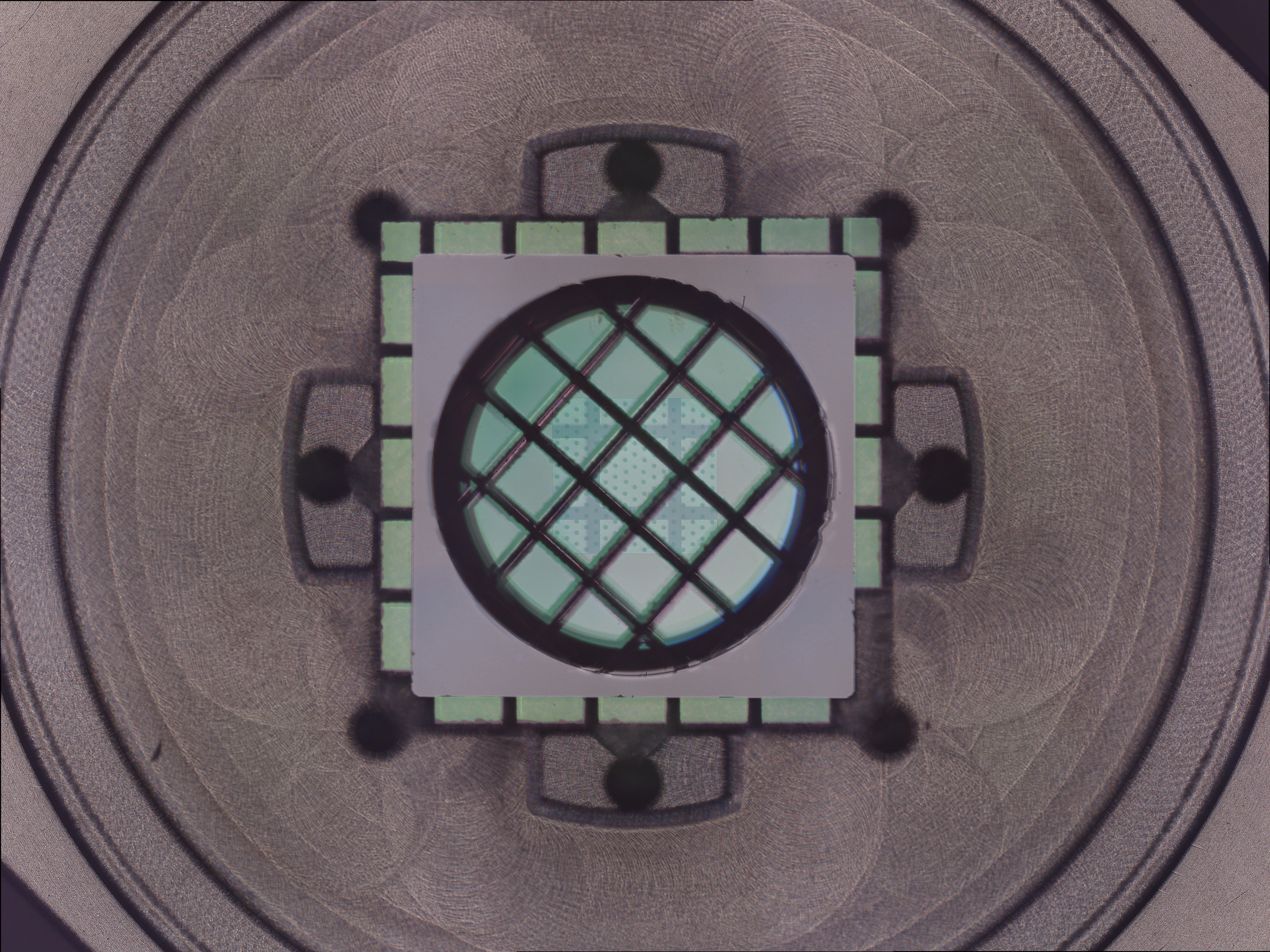
“The techniques we used to deal with notorious and complex noise sources are of high relevance and impact to the broader community of precision sensing and measurement,” says Guanhao Huang, one of the two PhD students leading the project.
The setup allowed the researchers to achieve “optical squeezing”, a quantum phenomenon where certain properties of light, like its intensity or phase, are manipulated to reduce the fluctuations in one variable at the expense of increasing fluctuations in the other, as dictated by Heisenberg’s principle.
By demonstrating optical squeezing at room temperature in their system, the researchers showed that they could effectively control and observe quantum phenomena in a macroscopic system without the need for extremely low temperatures.
The team believes the ability to operate the system at room temperature will expand access to quantum optomechanical systems, which are established testbeds for quantum measurement and quantum mechanics at macroscopic scales.
“The system we developed might facilitate new hybrid quantum systems where the mechanical drum strongly interacts with different objects, such as trapped clouds of atoms,” adds Alberto Beccari, the other PhD student leading the study. “These systems are useful for quantum information, and help us understand how to create large, complex quantum states.”
Written by EPFL
Image by Guanhao Huang & EPFL